Project Lead: Sierra Schleufer, Neuroscience
eScience Liaison: Bryna Hazelton
Humans experience remarkable visual acuity among mammals thanks to a retinal mosaic in which cone photoreceptors sensitive to three spans of the visual spectrum are increasingly concentrated toward the central visual field. Our trichromatic vision allows us to discriminate hues along two spectrally opponent axes in addition to luminance, thanks to an interleaved mosaic of L, M, and S cone photoreceptors sensitive to long-, middle-, and short-wavelength spans of the visual spectrum, respectively. Resolution and detection along these three axes are fundamentally limited by cone spatial and spectral topography (i.e. the ratio, density and arrangement of the 3 cones types in the photoreceptor mosaic). In some respects these arrangements are known to vary widely between people (e.g. the global ratio of L to M cones), with surprisingly little-to-no effect on standard color vision metrics, while other features are thought to be more consistent (e.g. percent S cones as a function of retinal eccentricity).
Our team explored how we can take advantage of extant analyses that are best suited to evaluate how cone arrangement influences and constrains spatial and color vision. We began by applying classic two-point correlation techniques to study the intra-cone spacing characteristics of L-, M-, and S- sub-mosaics within regions spanning the central ~5° radius of the retina in 4 human subjects (a classified human mosaic dataset of thus far unprecedented size in the field). Monte Carlo simulations were used to simulate cone mosaics with random spacing characteristics as a basis of comparison for the spatial arrangement of real cone data. For every cone sub-mosaic analyzed, a population of 1000 Uniform Monte Carlo mosaics were generated with cones randomly spaced within the rectangular bounds of the mosaic, which provided a distribution representative of uniform random arrangement. A second series of 1000 Monte Carlo mosaics were generated in which cones could only occupy actual positions of cones from the total mosaic (essentially shuffling the cone types of the real data). For each real and simulated mosaic, a matrix of inter-cone distances was calculated and represented as a histogram, then normalized by the mean of the uniform Monte Carlo histograms. Overlaying the real cone mosaic’s result on the mean +/- 2 std the two Monte Carlo distributions allows us to determine whether a cone-type sub-mosaic’s arrangement contains isotropic structure beyond what can be explained by occupying the hexagonally packed cone mosaic. The distribution of real intra-cone distances in the mosaic is assessed for whether cones are more spaced (crystalline) or close together (clumped) than cone-position-locked Monte Carlo distribution (as a function of distance from any given cone within the mosaic). We built this analysis into a fledgling package of open-source software tools designed to be highly modular, such that we (and, aspirationally, future collaborators) can expand our battery of analyses and datasets over time with minimal obstacle.
Preliminary results corroborate previous findings we’ve made using other analyses – that the central S-cone sub-mosaic features a degree of crystallinity statistically distinct from what we would see if the same number of S-cones randomly arranged within the cone mosaic. This suggests both that there are biological processes enforcing this spacing and an evolutionary pressure to do so. Meanwhile the L- and M- cone sub-mosaics are nearly completely indistinguishable from randomly arranged mosaics. However, additional work is required to estimate error within our classified cone mosaic data to fully interpret results like these. We will continue developing our analysis and the software framework with the goal of better understanding physiology that supports color vision. In the near future, this will include error estimation, assessment of inter-cone-type spacing characteristics, and analyses sensitive to anisotropic topography. Furthermore, we will continue to prioritize making these tools accessible and collaborative. This effort will produce the most detailed characterization of human spectral topography to date, in addition to bringing powerful techniques from other fields to retinal physiology. These findings will provide the basis for future experiments to test structure-to-function hypotheses regarding biophysical models and human psychophysics.
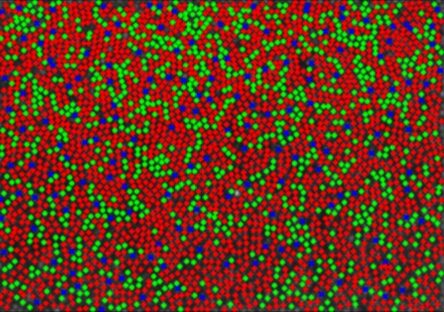